About
Why do we care about glaciers?
Glacier changes are recognized as one of the best natural indicators of climate change as glaciers only respond to more long-term climatic trends. The analysis of their changes through time is thus providing insights into past climatic fluctuations as well as on the impacts of current climate change. Glaciers are a major contributor to global sea-level rise and a precious resource of fresh water in many arid regions around the world (for human consumption and agriculture), a source of natural hazards, an important factor for hydro-power production and run-off, as well as for recreation and tourism. The monitoring of glacier changes is thus an important component of the global climate observing system (GCOS) and vital for many aspects of human life.
The field-based monitoring of selected glaciers started in 1894 with the regular observation of glacier length changes (or terminus fluctuations) and provides one of the longest climate data records we have. Since a few decades, satellite data provide globally more complete and complimentary information on glacier changes through time. In particular, changes in glacier extent and volume are mostly determined from satellite data these days, but also surface flow velocities are regularly derived for many of the 215,000 glaciers globally. These three variables (glacier extent, elevation change and velocities) are also investigated by the Glaciers CCI project.
An important dataset to spatially constrain calculations and determine glacier-specific changes is a globally complete glacier inventory, that provides outlines of each glacier in a vector format (shape file), supplemented by attribute information providing glacier characteristics. Such a dataset (the Randolph Glacier Inventory) was created by a major community effort in 2012 to improve global-scale calculations for IPCC AR5 and has been updated and improved several times since. The Glaciers CCI project and its pre-cursor GlobGlacier contributed substantially to this effort by providing glacier inventories for key regions.
A study (=> link) by Glaciers CCI team members analysed elevation changes across glaciers in High Mountain Asia (HMA) and revealed for the first time the high spatial variability of glacier response to climate change in this region. Subsequent studies revealed a very dense picture of very unusual glacier changes in the region. Our elevation change observations in HMA were complemented by local-scale to region-wide flow velocity maps, of which the latter are also derived in several other regions of the world for several points in time (e.g. Arctic Islands) using historic EO data. A related study revealed that flow velocities of numerous glaciers have significantly increased over the past decades, despite pre-dominantly negative mass balances.
Of particular interest these days are so-called surge-type glaciers that suddenly start to transport large amounts of mass from a higher reservoir zone towards the terminus, sometimes advancing over several kilometres in a few months or years. The erratic behaviour of these glaciers is still poorly understood, largely decoupled from climate forcing, related to natural hazards (outbursts of lakes that follow blocking of rivers) and contributing large amounts of ice mass to the ocean that are not yet properly accounted for in models determining future sea-level rise. There is thus some need to further strengthen research on this special type of glaciers, which is the main focus of the current Glaciers CCI+ project.
Data
The main products are glacier outlines and inventories, elevation and mass changes, and maps of flow velocities. They can be downloaded from a dedicated website hosted by ENVEO. Apart from creating datasets, a major effort has been and is spent on publications that are providing in-depth information about several of the produced datasets, the algorithms applied and the lessons learned. The studies range from change assessment over large regions to detailed investigations of individual glaciers. Below we shortly describe the methods used to generate the datasets. Further details can be found in the ATBD.
1. Glacier Outlines and Inventories
We are using multispectral optical satellite data (mostly Landsat and Sentinel-2) and a simple band ratio with a threshold to create binary maps of clean ice. This method works very well as glacier ice and snow have large differences in spectral reflectance in the visible and near infrared (VNIR) compared to the shortwave infrared (SWIR). The binary maps are converted from raster to vector format and are edited manually by visual inspection using a range of colour composites of the same image in the background. The main issues are that debris-covered ice is not mapped but should be included (omission errors) whereas ice bergs, sea ice or turbid water is included but should not (commission errors). For a better identification of debris-covered glaciers, we also consider coherence images from SAR sensors (ALOS PALSAR, Sentinel-1) that often reveal the moving parts of a glacier with extraordinary clarity.
Once outlines are corrected, they are digitally intersected with drainage divides derived by watershed modelling from a digital elevation model (DEM). The same DEM is finally used to determine for each glacier polygon a series of topographic parameters (e.g. minimum, maximum and median elevation, mean slope and aspect) as required for a glacier inventory. The finalized dataset is then submitted to the GLIMS glacier database. Current research has a focus on a more automated mapping of debris-covered glaciers and cloud-based processing of image stacks to avoid scene-by-scene processing and laborious mosaicking of results (e.g. in regions that are often cloud-covered).
Randolph Glacier Inventory version 5.0 - interactive globe
2. Elevation Changes
We utilize several methods to determine elevation changes of the glacier surface. These include repeat altimetry from optical (e.g. ICESat) and radar (e.g. Cryosat-2) sensors, comparison of altimetry data to a DEM (e.g. SRTM) or differencing of DEMs from two points in time (e.g. TanDEM-X – SRTM). Current techniques (=> link ) use stacks of multiple DEMs generated automatically from stereo sensors to derive more robust trends in elevation change for each DEM cell. Three critical points to consider before volume changes of entire glaciers can be derived from the datasets are (1) proper co-registration (a pre-processing step), (2) handling of artefacts and outliers and (3) spatial interpolation of data voids and uncovered regions (both post-processing steps). Whereas for issue (1) automated methods are in place (=> link ), issues (2) and (3) have to be solved individually by considering the highly variable nature of these issues. Many studies use filters (an elevation change threshold) to remove outliers and mean values of elevation changes as derived for specific elevation bands to extrapolate data voids (=> link).
However, these methods are not standardized so that different authors would obtain slightly different results for the same dataset. Another issue requiring attention is radar penetration when using DEMs obtained from InSAR (e.g. SRTM or TanDEM-X). Penetration into dry snow and firn varies with the band (e.g. is stronger for C than for X band) and the specific dielectric properties of the surface (e.g. moisture content). As the latter are generally unknown and can be locally highly variable, a standard correction might not provide the best results. Current research aims at a better determination of radar penetration, improved artefact handling and data void interpolation as well as completing global coverage.
3. Flow velocities
Glacier flow velocities can be derived automatically from repeat satellite imagery using offset tracking (optical & SAR) and interferometric techniques (SAR only). Synthetic Aperture Radar (SAR) data, over optical, has the advantage of being applicable all-year and in all-weather conditions. The InSAR technique has the highest accuracy, but requires satellite data from crossing orbits (e.g. ascending and descending) and usually works best only in comparably slow moving and flat terrain. The use of optical imagery requires visible surface features that can be tracked, so good contrast and limited self-similarity is essential. It is thus working better for ablation regions of glaciers than for snow-covered areas with little contrast. Unreliable data are filtered, causing data voids in the flow field that can partly be filled by processing several scene pairs and stacking them. Dynamically instable, small, fast flowing or surging glaciers are challenging for all techniques but are a key focus in this project.
Recent methodological advances and increased satellite coverage now allow for continuous retrieval of ice velocities over short periods (down to 6-12 days with Sentinel-1). This enables the monitoring of ice flow even on fast-flowing and surging glaciers, revealing precious insights into flow governing processes. Several studies by Glaciers CCI team members have investigated the related methods (=> link) and applied them to surging glaciers in HMA (=> link) or the Arctic (=> link). For the latter region SAR data are of particular importance as polar night and frequent cloud cover prevents continuous observations with optical sensors. The 6-day repeat period of Sentinel-1 is of special advantage as the short interval reduces decorrelation and provides more complete flow fields. Current methodological research has a focus on the combination of multiple sensors and a better consideration of smaller glaciers.
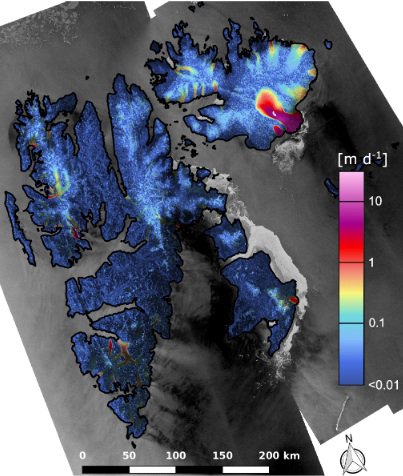
Figure 1: Glacier velocity in Svalbard derived from Sentinel-1 data. Image credit: contains modified Copernicus Sentinel-1 data (2016), processed by ENVEO.
Key Documents
Team
The Glaciers project is led by the Department of Geography, University of Zurich (GIUZ) as the prime contractor.
The EO team consists of four sub-contractors from four countries. Two of these are industry partners (Enveo, Gamma) and two are from Universities (GUIO, SEEL). The climate research group is supporting the project by providing advice, independent quality assessment and application of the generated datasets in glacier models. The key personnel of the consortium are:
Scientific Lead:
Dr. Frank Paul: Department of Geography, University of Zurich, Switzerland (GIUZ)
Project Manager:
Dr. Philipp Rastner: Department of Geography, University of Zurich, Switzerland (GIUZ)
EO Science Team:
Dr. Jan Wuite; Environmental Earth Observation, Innsbruck, Austria (ENVEO)
Dr. Thomas Nagler: Environmental Earth Observation, Innsbruck, Austria (ENVEO)
Prof. Dr. Andreas Kääb: Department of Geosciences, University of Oslo, Norway (GUIO)
Prof. Dr. Andrew Shepherd: School of Earth and Environment, University Of Leeds, United Kingdom (SEEL)
Dr. Tazio Strozzi: Gamma Remote Sensing AG, Gümligen, Switzerland (Gamma)
System Engineers (SE)
Dr. Andreas Wiesmann: Gamma Remote Sensing AG, Gümligen, Switzerland (Gamma)
Climate Research Group (CRG)
Prof. Michael Zemp: World Glacier Monitoring Service (WGMS), University of Zurich, Switzerland
Dr. Bruce Raup: Global Land Ice Measurements from Space (GLIMS), National Snow and Ice Data Center (NSIDC), Boulder (CO), USA
Dr. Liss M. Andreassen: Norwegian Water Ressources and Energy Directorate (NVE), Oslo, Norway
Prof. Ben Marzeion: Institute of Geography, University of Bremen, Bremen, Germany
Dr. Etienne Berthier: University of Toulouse, LEGOS-CNRS, Toulouse, France
Publications
Click on the following links for publications relating to the Glaciers project.
2023 |2022 |2021 |2020 |2019 | 2018 | 2017 | 2016 | 2015 | 2014 | 2013 | 2012 | 2011 |
Publications 2023
110. Berthier, E., Floricioiu, D., Gardner, A.S., Gourmelen, N., Jakob, L., Paul, F., Treichler, D., Wouters, B., Belart, J., Dehecq, A., Dussaillant, I., Hugonnet, R., Kääb, A., Krieger, L., Palsson, F. and Zemp, M. (2023): Measuring glacier mass changes from space - a review. Reports on Progress in Physics. 86, 036801, doi.org/10.1088/1361-6633/acaf8e.
109. Kääb, A. and Girod, L. (2023): Brief communication: Rapid ∼ 335 × 106 m3 bed erosion after detachment of the Sedongpu Glacier (Tibet), The Cryosphere, 17, 2533–2541.
108. Kääb, A., Bazilova, V., Leclercq, P.W., Mannerfelt, E.S. and Strozzi, T. (2023): Global clustering of recent glacier ̈surges from radar backscatter data, 2017–2022. J. Glaciol., doi.org/10.1017/ jog.2023.35.
107. Paul, F., Baumann, S., Anderson, B., and Rastner, P. (2023): Deriving a year 2000 glacier inventory for New Zealand from the existing 2016 inventory. Annals of Glaciology, 90.
Publications 2022
106. Hegglin, M. and 37 others (2022): Space-based Earth observations in support of the UNFCCC Paris Agreement. Frontiers in Environmental Science, 10, 941490.
105. Windnagel, A., Hock,R., Maussion, F., Paul, F., Rastner, P., Raup, B.H., and Zemp, M. (2022): Which glaciers are the largest in the world? Journal of Glaciology, 69 (274), 301-310.
104. Gärtner-Roer, I., Nussbaumer, S., Raup, B., Paul, F., Welty, E., Windnagel, A.K., Fetterer, F., Zemp, M. (2022): Democratizing glacier data – maturity of worldwide datasets and future ambitions. Frontiers in Climate, 4, 841103, doi.org/10.3389/fclim.2022.841103.
103. Paul, F., Piermattei L., Treichler D., Gilbert, L., Girod, L., Kääb, A., Libert, L., Nagler, T., Strozzi, T., Wuite, J. (2022): Three different glacier surges at a spot: what satellites observe and what not. The Cryosphere, 16, 2505–2526.
102. Tielidze, L. G., Nosenko, G. A., Khromova, T. E., Paul, F. (2022): Strong acceleration of glacier area loss in the Greater Caucasus over the past two decades. The Cryosphere, 16, 489-504.
Publications 2021
101. Leclercq, P.W., A. Kääb and B. Altena (2021): Brief Communication: Detection of glacier surge activity using cloud computing of Sentinel-1 radar data. The Cryosphere, 15, 4901–4907.
100. Shugar, D.H. and 52 others (2021): A massive rock and ice avalanche caused the 2021 disaster at Chamoli, Indian Himalaya. Science, 373 (6552), 300-306; doi.org/10.1126/science.abh4455.
99. Hugonnet R, McNabb R, Berthier E, Menounos B, Nuth C, Girod L, Farinotti D, Huss M, Dussaillant I, Brun F, Kääb A. (2021): Accelerated global glacier mass loss in the early twenty-first century. Nature, 592, 726-731; doi.org/10.1038/s41586-021-03436-z.
98. Kääb, A., Jacquemart, M., Gilbert, A., Leinss, S., Girod, L., Huggel, C., Falaschi, D., Ugalde, F., Petrakov, D., Chernomorets, S., Dokukin, M., Paul, F., Gascoin, S., Berthier, E. and Kargel, J. (2021): Sudden large-volume detachments of low-angle mountain glaciers - more frequent than thought. The Cryosphere, 15, 1751–1785; doi.org/10.5194/tc-15-1751-2021.
97. How, P., A. Messerli, E. Mätzler, M. Santoro, A. Wiesmann, R. Caduff, K. Langley, M.H. Bojesen, F. Paul, A. Kääb and J.L. Carrivick (2021): Greenland-wide inventory of ice marginal lakes using a multi-method approach. Scientific Reports, 11, 4481; doi.org/10.1038/s41598-021-83509-1.
Publications 2020
96. Altena, B. and Kääb, A. (2020): Ensemble matching of repeat satellite images applied to measure fast-changing ice flow, verified with mountain climber trajectories on Khumbu icefall, Mount Everest. Journal of Glaciology, 66 (260), 905-915.
95. Carturan, L., Rastner, P. and Paul, F. (2020): On the disequilibrium response and climate change vulnerability of the mass balance glaciers in the Alps. Journal of Glaciology. 66 (260), 1034-1050.
94. Goerlich, F., Bolch, T. and Paul, F. (2020): More dynamic than expected: An updated survey of surging glaciers in the Pamir. Earth Syst. Sci. Data, 12, 3161-3176.
93. Haga, O., McNabb, R., Nuth, C., Altena, B., Schellenberger, T. and Kääb, A. (2020). From high friction zone to frontal collapse: Dynamics of an ongoing tidewater glacier surge, Negribreen, Svalbard. Journal of Glaciology, 66 (259), 742-754.
92. Huber, J., McNabb, R. and Zemp, M. (2020). Elevation changes of west-central Greenland glaciers from 1985 to 2012 from remote sensing. Frontiers in Earth Science, 8, 35, doi.org/10.3389/feart.2020.00035
91. Paul, F. (2020): A 60-year chronology of glacier surges in the central Karakoram from the analysis of satellite image time-series. Geomorphology, 352, 106993.
90. Paul, F., Rastner, P., Azzoni, R.S., Diolaiuti, G., Fugazza, D., Le Bris, R., Nemec, J., Rabatel, A., Ramusovic, M., Schwaizer, G., and Smiraglia, C. (2020): Glacier shrinkage in the Alps continues unabated as revealed by a new glacier inventory from Sentinel-2. Earth Systems Science Data, 12(3), 1805-1821.
89. Popp, T., M.I. Hegglin, R. Hollmann, ... F. Paul, ... (2020): Consistency of satellite climate data records for Earth system monitoring. Bulletin American Meteorological Society, 101 (11), E1948-E1971.
88. Werder, M. A., Huss, M., Paul, F., Dehecq, A. and Farinotti, D. (2020): A Bayesian ice thickness estimation model for large-scale applications. Journal of Glaciology, 66 (255), 137-152.
87. Zemp, M., Huss, M., Eckert, N., Thibert, E., Paul, F., Nussbaumer, S.U. and Gärtner-Roer, I. (2020): Brief communication: Ad hoc estimation of glacier contributions to sea-level rise from latest glaciological observations. The Cryosphere, 14, 1043-1050.
Publications 2019
86. Altena, B., Scambos, T., Fahnestock, M., and Kääb, A. (2019): Extracting recent short-term glacier velocity evolution over Southern Alaska from a large collection of Landsat data, The Cryopshere, 13, 795-814.
85. Deschamps-Berger, C., C. Nuth, W. Van Pelt, E. Berthier, J. Kohler & B. Altena (2019): Closing the mass budget of a tidewater glacier, an example of Kronebreen, Svalbard. J. Glaciol., 65(249) 136-148.
84. Falaschi, D., Kääb, A., Paul, F., Tadono, T., Rivera, J.A., and Lenzano, L.E. (2019): Brief communication: Collapse of 4M m3 of ice from a cirque glacier in the Central Andes of Argentina. The Cryosphere, 13, 997-1004.
83. McNabb, R., Nuth, C., Kääb, A. and Girod, L. (2019): Sensitivity of geodetic glacier mass balance estimation to DEM void interpolation. The Cryosphere, 13, 895-910.
82. Nuth, C., A. Gilbert, A. Köhler, R. McNabb, T. Schellenberger, H. Sevestre, C. Weidle, L. Girod, A. Luckman and A. Kääb (2019): Dynamic vulnerability revealed in the collapse of an Arctic tidewater glacier. Scientific Reports, 9, 5541 (doi: 10.1038/s41598-019-41117-0).
81. Paul, F. (2019): Repeat glacier collapses and surges in the Amnye Machen mountain range, Tibet, possibly triggered by a developing rock-slope instability. Remote Sensing, 11(6), 708; doi: 10.3390/rs11060708.
80. Rastner, P., Notarnicola, C., Nicholson, L., Prinz, R., Sailer, R., Schwaizer, G. and Paul, F. (2019): Automated mapping of snow cover on glaciers and calculation of snow line altitudes from multi-temporal Landsat data. Remote Sensing, 11, 1410; doi: 10.3390/rs11121410.
79. Reinthaler, J., Paul, F., Delgado Granados, H., Rivera, A. and Huggel, C. (2019): Fast shrinkage of glaciers on active volcanoes in Latin America. Journal of Glaciology, 65 (252), 542-556.
78. Treichler, D., Kääb, A., Salzmann, N., and Xu, C.-Y. (2019): High Mountain Asia glacier elevation trends 2003–2008, lake volume changes 1990–2015, and their relation to precipitation changes. The Cryosphere, 13, 2977–3005.
77. Zemp, M., Huss, M., Thibert, E., Eckert, N., McNabb, R., Huber, J., Barandun, M., Machguth, H., Nussbaumer, S.U., Gärtner-Roer, I., Thomson, L., Paul, F., Maussion, F., Kutuzov, S., and Cogley, J.G. (2019): Global glacier mass changes and their contributions to sea-level rise from 1961 to 2016. Nature, 568, 382-386.
Publications 2018
76. Daiyrov M., C. Narama, T. Yamanokuchi, T. Tadono, A. Kääb and J. Ukita T. (2018): Regional geomorphological conditions related to recent changes of glacial lakes in the Issyk-Kul ba-sin, northern Tien Shan. Geosciences, 8(3), Art.no. 99, doi:10.3390/geosciences8030099.
75. Kääb, A., Leinss, S., Gilbert, A., Bühler, Y., Gascoin, S., Evans, S.G., Bartelt, P., Berthier, E., Brun, F., Chao, W., Farinotti, D., Gimbert, F., Guo, W., Huggel, C., Kargel, J.S., Leonard, G.J., Tian, L., Treichler D. and Yao, T. (2018): Massive collapse of two glaciers in western Tibet in 2016 after surge-like instability. Nature Geoscience, 11, 114–120.
74. Lindbäck, K., Kohler, J., Pettersson, R., Nuth, C., Langley, K., Messerli, A., Vallot, D., Matsuoka, K. and Brandt, O. (2018): Subglacial topography, ice thickness, and bathymetry of Kongsfjorden, northwestern Svalbard. Earth Syst. Sci. Data, 10, 1769-1781.
73. Mölg, N., Bolch, T., Rastner, P., Strozzi, T. and Paul, F. (2018): A consistent glacier inventory for the Karakoram and Pamir region derived from Landsat data: Distribution of debris cover and mapping challenges. Earth Systems Science Data, 10, 1807-1827.
72. Narama C., M. Daiyrov, M. Duishonakunov, T. Tadono, H. Sato, A. Kääb, J. Ukita, and K. Abdrakhmatov (2018): Large drainages from short-lived glacial lakes in the Teskey Range, Tien Shan Mountains, Central Asia . Nat. Hazards Earth Syst. Sci., 18, 983-995.
71. Robson, B.A., C. Nuth, P.R. Nielsen, L. Girod, M. Hendrickx and S.O. Dahl (2018): Spatial Variability in Patterns of Glacier Change across the Manaslu Range, Central Himalaya. Front. Earth Sci., 6, 12, doi: 10.3389/feart.2018.00012.
70. Sevestre, H., Benn, D. I., Luckman, A., Nuth, C., Kohler, J., Lindbäck, K. and Pettersson, R. (2018): Tidewater glacier surges initiated at the terminus. Journal of Geophysical Research: Earth Surface, 123, 1035–1051.
69. Winsvold S.H., A. Kääb, C. Nuth, L. Andreassen, W. Van Pelt, T. Schellenberger (2018): Using SAR satellite data time series for regional glacier mapping. The Cryosphere, 12, 867-890.
Publications 2017
68. Rastner, P., Strozzi, T. and Paul, F. (2017): Fusion of multi-source satellite data and DEMs to create a new glacier inventory for Novaya Zemlya. Remote Sensing, 9(11), 1122; doi: 10.3390/rs9111122.
67. Brun, F., E. Berthier, P. Wagnon, A. Kääb and D. Treichler (2017): A spatially resolved estimate of High Mountain Asia glacier mass balances from 2000 to 2016. Nature Geoscience, 10, 668-673; doi:10.1038/ngeo2999.
66. Girod, L., Nuth, C., Kääb, A., McNabb, R., and Galland, O. (2017): MMASTER: improved ASTER DEMs for elevation change. Remote Sensing, 9 (7), 704; doi:10.3390/rs9070704.
65. Merchant, C.J., F. Paul, et al. (2017): Uncertainty information in climate data records from Earth observation. Earth System Science Data, 9, 511-527; doi.org/10.5194/essd-9-511-2017.
64. Paul, F., T. Strozzi, T. Schellenberger and A. Kääb (2017): The 2015 surge of Hispar Glacier in the Karakoram. Remote Sensing, 9(9), 888; doi: 10.3390/rs9090888.
63. Strozzi, T., F. Paul, A. Wiesmann, T. Schellenberger and A. Kääb (2017): Circum-Arctic changes in the flow of glaciers and ice caps from satellite SAR data between the 1990s and 2017. Remote Sensing, 9(9), 947; doi: 10.3390/rs9090947
62. Altena, B. and A. Kääb (2017): Elevation change and improved velocity retrieval using orthorectified optical satellite data from different orbits. Remote Sensing, 9(3), 300; doi: 10.3390/rs9030300.
61. Farinotti, D., Brinkerhoff, D. J., Clarke, G. K. C., Fürst, J. J., Frey, H., Gantayat, P., Gillet-Chaulet, F., Girard, C., Huss, M., Leclercq, P. W., Linsbauer, A., Machguth, H., Martin, C., Maussion, F., Morlighem, M., Mosbeux, C., Pandit, A., Portmann, A., Rabatel, A., Ramsankaran, R., Reerink, T. J., Sanchez, O., Stentoft, P. A., Singh Kumari, S., van Pelt, W. J. J., Anderson, B., Benham, T., Binder, D., Dowdeswell, J. A., Fischer, A., Helfricht, K., Kutuzov, S., Lavrentiev, I., McNabb, R., Gudmundsson, G. H., Li, H., and Andreassen, L. M.: How accurate are estimates of glacier ice thickness? Results from ITMIX, the Ice Thickness Models Intercomparison eXperiment, The Cryosphere, 11, 949-970, doi: 10.5194/tc-11-949-2017.
60. Girod L., Nuth C., Kääb A., Etzelmüller B., and Kohler J. (2017). Terrain changes from images acquired on opportunistic flights by SfM photogrammetry. The Cryosphere, 11, 827-840, doi:10.5194/tc-11-827-2017.
59. Goerlich, F., T. Bolch, K. Mukherjee and T. Pieczonka (2017): Glacier Mass Loss during the 1960s and 1970s in the Ak-Shirak Range (Kyrgyzstan) from Multiple Stereoscopic Corona and Hexagon Imagery. Remote Sens. 2017, 9(3), 275; doi:10.3390/rs9030275.
58. Huber, J., A. Cook, F. Paul and M. Zemp (2017): A complete glacier inventory of the Antarctic Peninsula based on Landsat 7 images from 2000-2002 and other pre-existing data sets. Earth Systems Science Data, 9, 115-131, doi: 10.5194/essd-9-115-2017.
57. Kääb A., Altena B. and Mascaro J. (2017): Coseismic displacements of the 14 November 2016 Mw 7.8 Kaikoura, New Zealand, earthquake using the Planet optical cubesat constellation. Natural Hazards and Earth System Sciences, 17, 627-639, doi:10.5194/nhess-17-627-2017.
56. Köhler, A., Nuth, C.; Kohler, J., Berthier, E., Weidle, C. and Schweitzer, J. (2016): A 15 year record of frontal glacier ablation rates estimated from seismic data. Geophysical Research Letters. 43(23), 12155-12164 (doi: 10.1002/2016GL070589).
55. Marzeion, B., N. Champollion, W. Haeberli, K. Langley, P. Leclercq and F. Paul (2017): Observation of glacier mass changes on the global scale and its contribution to sea level change. Surveys in Geophysics, 38 (1), 105-130, doi: 10.1007/s10712-016-9394-y.
54. Narama C., M. Daiyrov, T. Tadono, M. Yamamoto, A. Kääb, R. Morita, J. Ukita (2017). Seasonal drainage of supraglacial lakes on debris-covered glaciers in the Tien Shan Mountains, Central Asia. Geomorphology, 286, 133-142, doi: 10.1016/j.geomorph.2017.03.002.
53. Strozzi T., A. Kääb and T. Schellenberger (2017): Frontal destabilization of Stonebreen, Edgeøya, Svalbard. The Cryosphere, 11, 553-566, doi: 10.5194/tc-11-553-2017.
52. Treichler D. and Kääb A. (2017): Snow depth from ICESat laser altimetry - A test study in southern Norway. Remote Sensing of Environment, 191, 389-401, doi: 10.1016/j.rse.2017.01.022.
Publications 2016
51. Kääb, A., Winsvold, S.H., Altena, B., Nuth, C., Nagler, T., Wuite, J. (2016): Glacier Remote Sensing Using Sentinel-2. Part I: Radiometric and Geometric Performance, and Application to Ice Velocity. Remote Sensing, 8(7), 598 (doi:10.3390/rs8070598)
50. Kronenberg, M., Barandun, M., Hoelzle, M., Huss, M., Farinotti, D., Azisov, E., Usubaliev, R., Gafurov, A., Petrakov, D. and Kääb, A.(2016): Mass balance reconstruction for Glacier No. 354, Tien Shan, from 2003- 2014. Annals of Glaciology, 57(71), 92-102.
49. Paul, F., S.H. Winsvold, A. Kääb, T. Nagler and G. Schwaizer (2016): Glacier Remote Sensing Using Sentinel-2. Part II: Mapping Glacier Extents and Surface Facies, and Comparison to Landsat 8. Remote Sens., 8(7), 575 (doi:10.3390/rs8070575).
48. Robson, B.A., D. Hölbling, C. Nuth, T. Strozzi and S.O. Dahl (2016): Decadal scale changes in glacier area in the Hohe Tauern National Park, Austria determined by object-based image analysis. Remote Sensing, 8, 67 (10.3390/rs8010067).
47. Winsvold, S., A. Kääb and C. Nuth (2016): Regional glacier mapping using optical satellite data time series. JSTARS (doi: 10.1109/JSTARS.2016.2527063).
Publications 2015
46. Allison, I., Colgan, W., King, M., Paul, F. (2015): Ice sheets, glaciers and sea level. In: Haeberli, W. and C. Whiteman, eds. Snow and Ice-Related Hazards, Risks, and Disasters, Amsterdam, Netherlands, Elsevier, 714-748.
45. Bolch, T. (2015): Glacier area and mass changes since 1964 in the Ala Archa Valley, Kyrgyz Ala-Too, northern Tien Shan. Лёд и Снег (Ice and Snow) 129(1): 28-39.
44. Holzer, N., S. Vijay, T. Yao, B. Xu, M. Buchroithner and T. Bolch(2015): Four decades of glacier variations at Muztagh Ata (eastern Pamir): a multi-sensor study includ-ing Hexagon KH-9 and Pléiades data. The Cryosphere, 9, 2071-2088.
43. Kääb, A., Treichler D., Nuth C. and Berthier E. (2015): Brief Communication: Contending estimates of 2003–2008 glacier mass balance over the Pamir–Karakoram–Himalaya. The Cryosphere, 9, 557- 564. doi:10.5194/tc-9-557-2015
42. Le Bris, R. and F. Paul (2015): Glacier-specific elevation changes in western Alaska. Annals of Glaciology, 56 (70), 184-192.
41. Nuth, C., Hagen, J.O., and Kohler, J. (2015): Ch 4. Glaciers in Geoscience Atlas of Svalbard (ed. Dallmann W.K.). Norsk Polarinstitutt Rapport; 148, Tromsø.
40. Paul, F. and 24 others (2015): The Glaciers Climate Change Initiative: Algorithms for creating glacier area, elevation change and velocity products. Remote Sensing of Environment, 162, 408-426. doi:10.1016/j.rse.2013.07.043
39. Paul, F. (2015): Revealing glacier flow and surge dynamics from animated satellite image sequences: examples from the Karakoram. The Cryosphere, 9, 2201-2214.
38. Paul, F. (2015): Kartierung von Gletschern mit Satellitendaten und das globale Gletscherinventar. In: Lozán, J.L., H. Grassl, D. Kasang, D. Notz, and H. Escher-Vetter (Hrsg.): Warnsignal Klima: Das Eis der Erde (Kap. 4.1), 103-110.
37. PelliCCIotti, F., Stephan, C., Miles, E., Herreid, S., Immerzeel, W., Bolch, T. (2015): Mass-balance changes of the debris-covered glaciers in the Langtang Himal, Nepal, between 1974 and 1999. Journal of Glaciology 61(226): 373-386, doi: 10.3189/2015JoG13J237
36. Pieczonka, T., Bolch, T. (2015): Region-wide glacier mass budgets and area changes for the Central Tien Shan between ~1975 and 1999 using Hexagon KH-9 imagery. Global and Planetary Change, 128, 1-13. 10.1016/j.gloplacha.2014.11.014.
35. Raup, B.H., L.M. Andreassen, T. Bolch and S. Bevan (2015): Remote sensing of glaciers. In: Tedescco, M. (ed.): Remote Sensing of the Cryosphere, John Wiley & Sons, 123-165.
34. Robson, B.A., C. Nuth, S.O. Dahl, D. Hölbling, T. Strozzi and P. R. Nielsen (2015): Automated classification of debris-covered glaciers combining optical, SAR and topo-graphic data in an object-based environment. Remote Sensing of Environment, 170, 372-387.
33. Schellenberger, T., T. Dunse, A. Kääb, J. Kohler, C. H. Reijmer: Surface speed and frontal ablation of Kronebreen and Kongsbreen, NW-Svalbard, from SAR offset tracking. The Cryosphere, 9, 2339-2355.
32. Shangguan, D. H., Bolch, T., Ding, Y. J., Kröhnert, M., Pieczonka, T., Wetzel, H. U., Liu, S. Y. (2015): Mass changes of Southern and Northern Inylchek Glacier, Central Tian Shan, Kyrgyzstan, during ∼1975 and 2007 derived from remote sensing data, The Cryosphere 9: 703-717, doi:10.5194/tc-9-703-2015.
31. Wang, D. and A. Kääb (2015): Modeling glacier elevation change from DEM time series. Remote Sensing, 7(8), 10117-10142.
30. WGMS (2015): Global Glacier Change Bulletin No. 1 (2012–2013). M. Zemp, I. Gärtner-Roer, S. Nussbaumer, F. Hüsler, H. Machguth, N. Mölg, F. Paul and M. Hoelzle (eds.), ICSU(WDS)/IUGG(IACS)/ UNEP/UNESCO/WMO, World Glacier Monitoring Service, Zurich, Switzerland, 230 pp.
29. Zemp, M., H. Frey, I. Gärtner-Roer, S.U. Nussbaumer, M. Hoelzle, F. Paul, W. Haeberli, et al. (2015): Historically unprecedented global glacier changes in the early 21st century. Journal of Glaciology, 61 (228),745-762.
Publications 2014
28. Bolch, T. (2015): Glacier area and mass changes since 1964 in the Ala Archa Valley, Kyrgyz Ala-Too, northern Tien Shan. Лёд и Снег (Ice and Snow) 129(1): 28-39. doi: 10.15356/2076-6734-2015-1-28-39
27. Frey, H., Machguth, H., Huss, M., Huggel, C., Bajracharya, S., Bolch, T., Kulkarni, A., Linsbauer, A., Salzmann, N., and Stoffel, M. (2014): Ice volume estimates for the Himalaya–Karakoram region: evaluating different methods, The Cryosphere 8: 2313-2333.
26. Kargel, J.S., Leonard, G.J., Bishop, M.P., Kääb, A., Raup, B.H. (Eds.) (2014): Global Land Ice Measurements from Space. Springer Praxis Books, 876 pp. (link: http://link.springer.com/book/10.1007%2F978-3-540-79818-7)
25. Neckel, N., Kropacek, J., Bolch, T., Hochschild, V. (2014): Glacier elevation changes on the Tibetan Plateau between 2003 – 2009 derived from ICESat measurements Environmental Research Letters 9: 014009 (7pp), doi:10.1088/1748-9326/9/1/014009.
24. Paul, F. and Mölg, N. (2014): Hasty retreat of glaciers in northern Patagonia from 1985 to 2011. Journal of Glaciology, 60 (224), 1033-1043. doi:10.3189/2014JoG14J104
23. Pfeffer, W.T., A.A. Arendt, A. Bliss, T. Bolch, J.G. Cogley, A.S. Gardner, J.-O. Hagen, R. Hock, G. Kaser, C. Kienholz, E.S. Miles, G. Moholdt, N. Mölg, F. Paul, V. Radic, P. Rastner, B.H. Raup, J. Rich, M.J. Sharp and the Randolph Consortium (2014): The Randolph Glacier Inventory: a globally complete inventory of glaciers. Journal of Glaciology 60(211): 537-552. doi: 10.3189/2014JoG13J176.
22. Rastner, P., Bolch, T., Notarnicola, C., Paul, F. (2014): A comparison of pixel- and object based glacier classification with optical satellite images. IEEE Journal of Selected Topics of Applied Earth Observation, 7(3): 853-862, doi: 10.1109/JSTARS.2013.2274668.
Publications 2013
21. Bhambri, R., Bolch, T., Kawishwar, P., Dobhal, D.P., Srivastava, D., Pratap, B. (2012): Heterogeneity in glacier response in the Shyok valley, northeast Karakoram. The Cryosphere 7: 1384-1398.
20. Bolch, T., Sandberg Sørensen, L., Simonssen, S.B., Mölg, N., Machguth, H., Rastner, P., Paul, F. (2013): Mass loss of Greenland’s glaciers and ice caps 2003-2008 revealed from ICESat laser altimetry data. Geophysical Research Letters 40, doi: 10.1029/2012GL054710.
- Gardelle, J., Berthier, E., Arnaud, Y., Kääb, A.: Region-wide glacier mass balances over the Pamir-Karakoram-Himalaya during 1999–2011, The Cryosphere 7, 1263-1286.
- Gardner, A. S., Moholdt, G., Cogley, J. G., Wouters, B., Arendt, A. A., Wahr, J., Berthier, E., Pfeffer, T. W., Kaser, G., Hock, R., Ligtenberg, S. R. M., Bolch, T., Sharp, M.J., Hagen, J. O., van den Broeke, M. R., Paul, F.: (2013): A reconciled estimate of glacier contributions to sea-level rise: 2003 to 2009. Science 340: 852–857.
- Hollmann, R., Merchant, C., Saunders, R., Downy, C., Buchwitz, M., Cazenave, A., Chuvieco, E., Defourny, P., de Leeuw, G., Forsberg, R., Holzer-Popp, T., Paul, F., Sandven, S., Sathyendranath, S., van Roozendael, M., Wagner, W. (2013): The ESA Climate Change Initiative: satellite data records for essential climate variables. Bulletin of the American Meteorological Society, 94(10): 1541-1552.
- Nuth, C., Kohler, J., König, M., von Deschwanden, A., Hagen, J. O., Kääb, A., Moholdt, G., and Pettersson, R. (2013): Decadal changes from a multi-temporal glacier inventory of Svalbard, The Cryosphere, 7, 1603-1621, doi:10.5194/tc-7-1603-2013.
- Pieczonka, T., Bolch, T., Wei, J., Liu, S. (2013): Heterogeneous mass loss of glaciers in the Aksu-Tarim Catchment (Central Tien Shan) revealed by 1976 KH-9 Hexagon and 2009 SPOT-5 stereo imagery. Remote Sensing of Environment 130, 233-244.
- Paul, F., Barrand, N., Berthier, E., Bolch, T., Casey, K., Frey, H., Joshi, S.P., Konovalov, V., Le Bris, R., Mölg, N., Nosenko, G., Nuth, C., Pope, A., Racoviteanu, A., Rastner, P., Raup, B., Scharrer, K., Steffen, S., Winsvold, S. (2013): On the accuracy of glacier outlines derived from remote sensing data. Annals of Glaciology54(63), 171-182.
Publications 2012
- Arendt, A. et al. (2012): Randolph Glacier Inventory [v2.0]: A data set of Global Glacier Outlines, Boulder, Colorado, Digital Media.
- Andreassen, L.M., Winsvold, S.H., Paul, F., Hausberg, J.E. (2012): Inventory of Norwegian Glaciers. Norwegian Water Resources and Energy Directorate, Rapport 38-2012, 240 pp.
- Bolch, T., Kulkarni, A., Kääb, A., Huggel, C., Paul, F., Cogley, G., Frey, H., Kargel, J.S., Fujita, K., Scheel, M., Bajracharya, S., Stoffel, M. (2012): The state and fate of Himalayan glaciers. SCIENCE 336(6079), 310–314.
- Debella-Gilo, M.; Kääb, A. (2012) Measurement of Surface Displacement and Deformation of Mass Movements Using Least Squares Matching of Repeat High Resolution Satellite and Aerial Images. Remote Sensing 4(1), 43-67.
- Debella-Gilo M.; Kääb, A. (2012): Locally adaptive template sizes for matching repeat images of Earth surface mass movements. ISPRS J. Photogramm. Remote Sens. 69, 10-28.
- Heid, T., Kääb, A. (2012): Evaluation of existing image matching methods for deriving glacier surface displacements globally from optical satellite imagery. Remote Sensing of Environment 118, 339-355.
- Heid T., Kääb A. (2012): Repeat optical satellite images reveal widespread and long term decrease in land-terminating glacier speeds. The Cryosphere 6, 467-478.
- Kääb A. , Berthier, E., Nuth, C., Gardelle, J., Arnaud. Y. (2012): Contrasting patterns of early twenty-first-century glacier mass change in the Himalayas. Nature 488(7412), 495-498.
- Leclercq, P.W., Weidick, A., Paul, F., Bolch. T., Citterio, M., Oerlemans, J. (2012): Historical glacier length changes in West Greenland. The Cryosphere 6, 1339-1343.
- Nuth, C.; Schuler, T.; Kohler, J.; Altena, B.; Hagen, J. (2012): Estimating the long-term calving flux of Kronebreen, Svalbard, from geodetic elevation changes and mass-balance modelling. Journal of Glaciology 58(207), 119-133.
- Paul, F., Bolch, T., Kääb, A., Nagler, T., Shepherd, A., Strozzi, T.(2012): Satellite-based glacier monitoring in the ESA Project Glaciers_CCI. Proceedings of the IGARSS Conference, 23.-27.7.2012, Munich, Germany: 3222-3225.
- Rastner, P., Bolch, T., Mölg, N., Machguth, H., Paul, F. (2012): The first complete glacier inventory for the whole of Greenland. The Cryosphere 6, 1483-1495.
- Shepherd, A., & et al. (2012). A reconciled estimate of ice-sheet mass balance. Science, 338, 1183–1189.
Related links
Global Terrestrial Network for Glaciers (GTN-G)
World Glacier Monitoring Service (WGMS)
Global Land Ice Measurements from Space (GLIMS)
Randolph Glacier Inventory (RGI)
International Association for the Cryospheric Sciences (IACS)
IACS Working Group on the RGI and its role in future glacier monitoring and GLIMS
News and events
Contacts
Science lead: Frank Paul (frank.paul@geo.uzh.ch)
Latest news & events
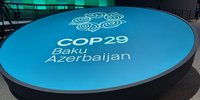
ESA at COP29
ESA is participating in COP29 to highlighting satellites' role in tackling climate change
Mehr erfahren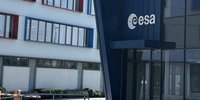
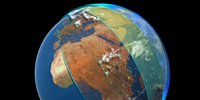
Call for new projects: Additional Essential Climate Variables
New R&D procurement as part of ESA's CLIMATE-SPACE programme
Mehr erfahren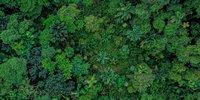
Open Competitive Tender for CLIMATE-SPACE Knowledge Exchange
ESA Tender Action Number: 1-12141. ESA Activity Number: 1000039650.
Mehr erfahren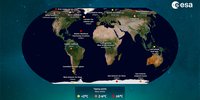
Satellites a ‘clear opportunity’ for forewarning of climate tipping points
New research offers guidance on how remote sensing can help understand and detection tipping systems
Mehr erfahren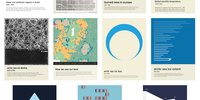
Little Pictures winner announced at COP28
Results of Europe-wide climate data visualisation showcased
Mehr erfahren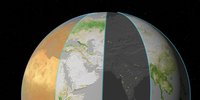
New Tender: CROSS-ECV ACTIVITIES Tender Action Number: 1-12062
New tender issued by the ESA Climate Office (Activity Number: 1000039196)
Mehr erfahren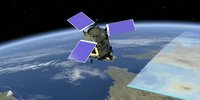
Harnessing Earth Observation for Climate Action
ESA in conversation Prof Jim Skea with IPCC Chair during the COP28 Earth Information day
Mehr erfahren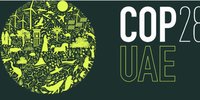
COP28: ESA Climate Office events
The Climate Office is contributing to several events at COP28, UAE, Dubai
Mehr erfahren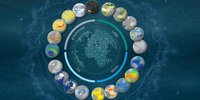
Taking climate action with Earth observation
Satellites' contribution to understanding climate change and supporting climate action are under the COP28 spotlight
Mehr erfahren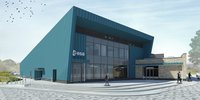
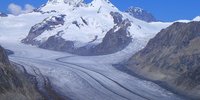
Global glacier surges mapped from space
Glacier surge events have been mapped on global-scale using Sentinel-1 and -2 research
Mehr erfahren